Purpose and objectives of the project and/or research question
The goal of this pilot project is to date the formation of various types of structures and fault rocks and to constrain their role and impact on deformation and on the location of the next large earthquake along the southern portion of the San Andreas fault (SAF) in the Coachella Valley (Fig. 1).
Main hypothesis: the SAF fault system in the Coachella Valley exhibits increased locking northwestwards from Durmid Hill and the Mecca Hills in the southeast, where it is locked into tight ladder structures, to the Indio Hills in the northwest (Fig. 2), which corresponds to an early-stage, evolving ladder structure located at the intersection of the tectonically active Landers–Mojave Line and the SAF. The SAF has one of the highest probabilities of a future large earthquake in California (Field et al., 2015).
Background and overview of current research
Despite decades of studying major fault zones, predicting the location and magnitude of future earthquakes eludes geoscientists. The project targets the southern portion of the SAF in California in the Coachella Valley, where the SAF switches from transpressional to trans-tensional settings southwards and where the next large earthquake in California is expected to take place (e.g., Crowell and Sylvester, 1979; Janecke et al., 2018; Koehl et al., 2023; Fig. 2).
Thus far, recent movements along the SAF are generally estimated by dating offset features such as fans and channels using U–Pb dating of pedogenic carbonate clast coating and/or cosmogenic isotope dating of such features (e.g., Keller et al., 1982; van der Woerd et al., 2006; Gray et al., 2014; Blisniuk et al., 2021). However, such structures are not always available because (1) they were eroded (e.g., due to subsequent uplift), or (2) did not have time to form between two major episodes of slip, thus casting a time-resolution limitation on the method and associated geochronological ages.
A more direct way of dating movement and earthquake occurrences is to date fault rocks. This was done along the locked and creeping northern portion of the fault (e.g., Russell et al., 1989; Coffey et al., 2022), but is still lacking for the locked southern portion of the SAF in the Coachella Valley (Fig. 1). Moreover, these studies did not relate the ages obtained to specific structure types (e.g., SAF-parallel faults vs SAF-oblique cross faults, main SAF, major SAF splay, etc.). Locked portions of the fault are more exposed to large earthquakes due to their inability to release stress through frequent aseismic creep events.
In addition, some studies have suggested that the SAF in the Coachella Valley is particularly at risk of a major earthquake because movement in the north is partly accommodated by the eastern California shear zone, whereas movement in southern California is mostly accommodated by the SAF (e.g., Dolan et al., 2007; Figs. 1 & 2). Furthermore, recent studies in the Coachella Valley have shown that deformation along the SAF has gradually tightened in the past 3 million years, resulting in the formation of complex structures such as ladder, which include oblique cross faults and folds (Janecke et al., 2018; Bergh et al., 2019), and that the recent Mw ≥ 5.0 earthquakes along the Landers–Mojave Line have been consistently converging towards the intersection of the SAF and the eastern California shear zone in the Indio Hills (Nur et al., 1993a, 1993b; Koehl et al., 2022a; Fig. 2).
We propose to date the formation of syn-kinematic minerals along the main SAF and related cross faults in the Coachella Valley to map the frequency of major movements and earthquakes along this segment of the fault and evaluate the role of ladder structures in locking major strike-slip faults and localizing earthquakes. Of particular interest is the role and timing of formation of (1) cross faults mapped by Janecke et al. (2018), Bergh et al. (2019), and Koehl et al. (2022a) respectively in Durmid Hill, Mecca Hills, and Indio Hills, which strike oblique to the main SAF, and (2) dextral freeway junctions (Platt and Passchier, 2016), which occur where subparallel dextral faults merge with the SAF (e.g., Indio Hills fault – Koehl et al., 2022a, and Skeleton Canyon fault – Bergh et al., 2019; Fig. 2).
Cross faults are suspected to reflect a gradual tightening and locking of SAF fault segments into ladder structures at zones of overlap with adjacent, subparallel major faults (Janecke et al., 2018; Bergh et al., 2019), whereas freeway junctions between major dextral strike-slip fault may lead tp counterclockwise block rotation.
Proposed research design (materials and methods)/project plan
The project will collect detailed structural measurements and samples of fault rocks (e.g., fault gouge) and folded rocks (in fine-grained rocks) at stepover and zones of overlap of the main NW–SE-striking fault segments of the SAF and related adjacent faults in the Indio Hills, Mecca Hills, and Durmid Hill during structural fieldwork (M1; Sylvester and Smith, 1976; Bürgmann, 1991; Janecke et al., 2018; Bergh et al., 2019; Koehl et al., 2022a). Notably, NE–SW- to E–W-striking cross faults will be sampled to test whether they formed prior to, together with, or after the main SAF. The folded, hematite-coated fault gouge along the main segment of the SAF (Bergh et al., 2019) and synkinematic calcite and hematite along the Skeleton Canyon fault, a major splay of the SAF, in the Mecca Hills, are high-priority targets.
Further suitable localities for sampling with good outcrop exposures will be identified using satellite images (Google Earth) prior to fieldwork. The collected fault rock samples will be thin-sectioned (M2) and analyzed using optical microscopy and Scanning Electron Microscopy to identify syn-kinematic minerals for K–Ar, U–Pb, U–Th–He, and/or U series/U–Th geochronological analyzes (M3–4). K–Ar geochronology will date the formation of syn-kinematic illite and/or illite–smectite in fault gouge and fold structures (University of Göttingen, Germany). U–Pb (carbonates, apatite), U–Th–He (apatite, hematite), and U series/U–Th (carbonates) analyses will be performed at the Noble Gas Thermochronometry Center and Uranium Daughter Laboratory at the Berkeley Geochronology Center and paid by the Berkeley Geochronology Center.
U–Pb and K–Ar analyses will be used to date several to tens of million years old movement events (e.g., Coffey et al., 2022), while the high-precision (< 1% error) U series will yield information on recent (1–100 ka old) events (e.g., Ambers, 2005). Such datasets are still lacking for the main SAF and related splays (e.g., Indio Hills fault, Skeleton Canyon fault, Eastern Shoreline fault), but are available for nearby faults in the Mecca Hills (e.g., Painted Canyon fault; Moser et al., 2017; DiMonte et al., 2022).
Expected outcomes and impact
The project will provide new constraints on the recent tectonic evolution of the SAF. Key constraints will be obtained on which fault moved, when, and how frequently, and yield information about the role of specific types of structures in localizing deformation and accumulating stress along major strike-slip faults (e.g., cross faults, en echelon folds, ladder structures, freeway junctions). The new time constraints obtained during the project will also contribute to setting the Coachella Valley and southern California into a broader, regional perspective and allow regional correlation of fault movements along the margin. This should in turn facilitate plate tectonics reconstructions along the southwestern margin of North America in the Cenozoic.
The newly tested workflow, including structural fieldwork and the use of multiple geochronometers (K–Ar, U–Pb, U–Th–He, and U series) will help predict the location of future large earthquakes along major faults in populated areas and contribute to mitigate disaster risk.
This is connected to the United Nations’ sustainable development goal 11 (sustainable cities and communities). Moreover, the project will collaborate with the ArcTec project (PI: Dr. Koehl), which is funded by the European Commission and focuses on newly identified, deeply buried, thousands of kilometres long faults in the Norwegian Arctic that formed c. 600 million years ago (Koehl et al., 2022b). These were repeatedly reactivated as strike-slip faults and are the most active faults in Norway at present as suggested by recent earthquake cycles (Koehl and Rimando, 2023 submitted).
In addition, the faults show comparable geometries and kinematics to the SAF in California, e.g., hundreds of kilometres wide left bends like the Big Bend, ladder structures, and strike-slip kinematics. The SAF, therefore, represents an outstanding analogue to the faults in Norway. The proposed project will notably provide detailed field characterization of mesoscale structures and information on the role and kinematics of various types of structures along major, active strike-slip faults. Meso-scale structures along the faults in Norway are especially difficult to infer because the faults are buried deeply under several kilometres of sediments.
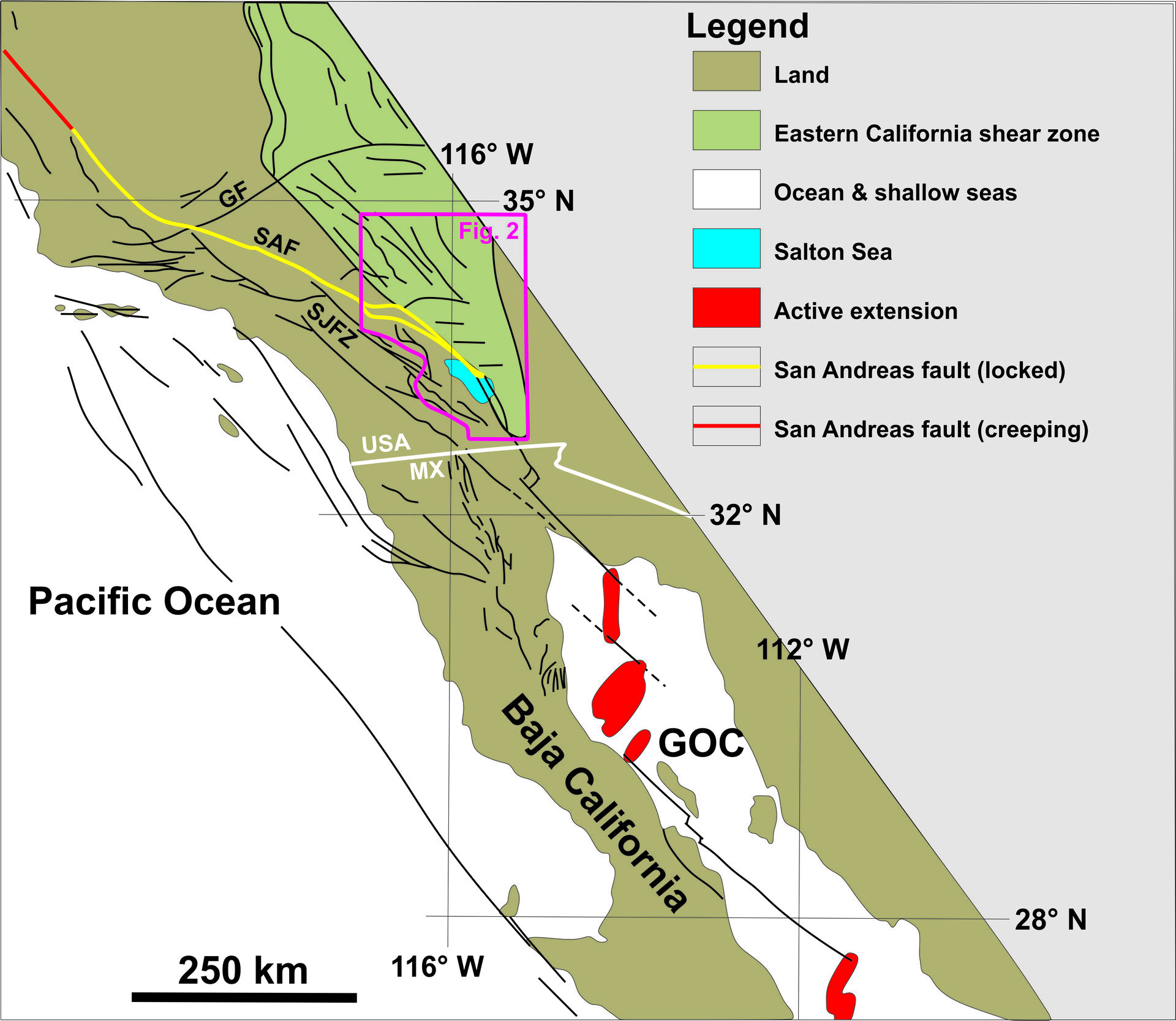
Click here for a bigger version.
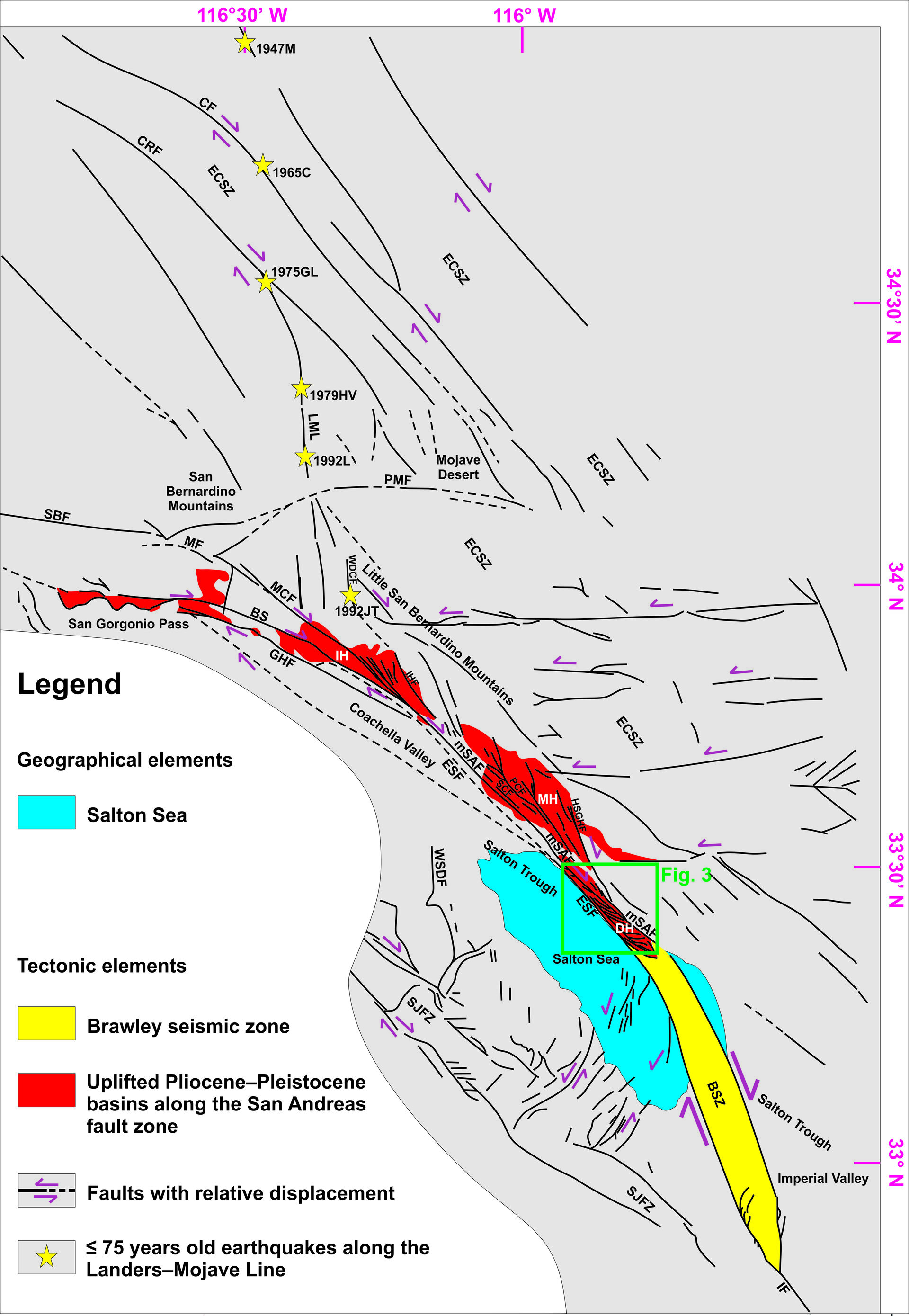
Abbreviations: 1947M: 1947 Manix earthquake; 1965C: 1965 Calico earthquake; 1975GL: 1975 Galway Lake earthquake; 1979HV: 1979 Homestead Valley earthquake; 1992JT: 1992 Joshua Tree earthquake; 1992L: 1992 Landers earthquake; BS: Banning strand; BSZ: Brawley seismic zone; CF: Calico fault; CRF: Camp Rock fault; DH: Durmid Hill uplift; ECSZ: eastern California shear zone; ESF: East Shoreline fault; GHF: Garnet Hill fault; HSGHF: Hidden Springs–Grotto Hills fault; IF: Imperial fault; IH: Indio Hills uplift; IHF: Indio Hills fault; LML: Landers–Mojave Line; MCF: Mission Creek fault; MF: Mill Creek fault; MH: Mecca Hills uplift; mSAF: main San Andreas fault; PCF: Painted Canyon fault; PMF: Pinto Mountain fault; SBF: San Bernardino fault; SCF: Skeleton Canyon fault; SJFZ: San Jacinto fault zone; WDCF: West Deception Canyon fault; WSDF: West Salton detachment fault.
Click here for a bigger version.
References
Ambers, J.: Archaeometry and antique analysis, Dating of artifacts, in: Encyclopedia of Analytical Science, edited by: Worsfold, P., Townshend, A. and Poole, C., Chemistry, Molecular Science and Chemical Engineering, 2nd edition, 109–117, 2005. Bergh, S. G., Sylvester, A. G., Damte, A. and Indrevær, K.: Polyphase kinematic history of transpression along the Mecca Hills segment of the San Andreas fault, southern California, Geosphere, 15, 34 pp., 2019.Blisniuk et al., 2021. Bürgmann, R.: Transpression along the southern San Andreas fault, Durmid Hill, California, Tectonics, 10, 6, 1152–1163, 1991. Coffey, G. L., Savage, H. M., Polissar, P. J., Cox, S. E., Hemming, S. R., Winckler, G. and Bradbury, K. K.: History of earthquakes along the creeping section of the San Andreas fault, California, USA, Geology, 50, 4, 516–521, 2022. Crowell, J. C. and Sylvester, A. G.: Tectonics of the juncture between the San Andreas fault system and the Salton trough, southeastern California, Geological Society of America Annual Meeting, San Diego, California, USA, edited by: Crowell, J. C. and Sylvester A. G., University of California Santa Barbara, California, USA, 195 pp., 1979. DiMonte, A. A., Ault, A. K., Hirth, G. and Bradbury, K. K.: Hematite accommodated shallow, transcient Pleistocene slow slip in the exhumed southern San Andreas fault system, California, USA, Geology, 50, 12, 1443–1447, 2022. Dolan, J. F., Bowman, D. D. and SAmmis, C. G.: Long-range and long-term fault interactions in Southern California, Geology, 35, 9, 855–858, 2007. Field, E. H., Biasi, G. P., Bird, P., Dawson, T. E., Felzer, K. R., Jackson, D. D., Johnson, K. M., Jordan, T. H., Madden, C., Michael, A. J., Milner, K. R., Page, M. T., Parsons, T., Powers, P. M., Shaw, B. E., Thatcher, W. R., Weldon II, R. J. and Zeng, Y.: Long-Term Time-Dependent Probabilities for the Third Uniform California Earthquake Rupture Forecast (UCERF3), Bulletin of Seismological Society of America, 105, 2A, 511–543, 2015. Gray, H. J., Owen, L. A., Dietsch, C., Beck. R. A., Caffee, M. A., Finkel, R. C. and Mahan, S. A.: Quaternary landscape development, alluvial fan chronology and erosion of the Mecca Hills at the southern end of the San Andreas Fault Zone, Quaternary Science Reviews, 105, 66–85, 2014. Janecke, S. U., Markowski, D. K., Evans, J. P., Persaud, P. and Kenney, M.: Durmid ladder structure and its implications for the nucleation sites of the next M >7.5 earthquake on the San Andreas fault or Brawley seismic zone in southern California, Lithosphere, 10, 5, 602–631, 2018. Keller, E. A., Bonkowski, M. S., Korsch, R. J. and Schlemon, R. J.: Tectonic geomorphology of the San Andreas fault zone in the southern Indio Hills, Coachella Valley, California, GSA Bull., 93, 46–56, 1982. Koehl, J.-B. P. and Rimando, J.: Recent earthquake sequences around Svalbard associated with reactivated–overprinted Timanian thrust systems, Norwegian Journal of Geology, 2023 submitted. Koehl, J.-B. P., Bergh, S. G. and Wemmer, K.: Neoproterozoic and post-Caledonian exhumation and shallow faulting in NW Finnmark from K–Ar dating and p/T analysis of fault rocks, Solid Earth, 9, 923–951, 2018. Koehl, J.-B. P., Bergh, S. G. and Sylvester, A. G.: Tectonic evolution of the Indio Hills segment of the San Andreas fault in southern California, southwestern USA, Solid Earth, 13, 1169–1190, 2022a. Koehl, J.-B. P., Magee, C. And Anell, I. M.: Impact of Timanian thrust systems on the late Neoproterozoic–Phanerozoic tectonic evolution of the Barents Sea and Svalbard, Solid Earth, 13, 85–115, 2022b. Koehl, J.-B. P., Bergh, S. G. and Sylvester, A. G.: From transtension to transpression and implications of the next Big One along the San Andreas fault, 16th February 2023, Weekly Seminar, University of Portsmouth, Portsmouth, UK, 2023. Moser, A. C., Evans, J. P., Ault, A. K., Janecke, S. U. and Bradbury, K. K.: (U–Th)/He thermochronometry reveals Pleistocene punctuated deformation and synkinematic hematite mineralization in the Mecca Hills, southernmost San Andreas Fault zone, Earth and Planetary Science Letters, 476, 87–99, 2017. Nur, A., Hagai, R. and Beroza, G. C.: The Nature of the Landers-Mojave Earthquake Line, Science, 261, 201–203, 1993a. Nur, A., Hagai, R. and Beroza, G. C.: Landers-Mojave earthquake Line: A New Fault System?, GSA Today, 3, 10, 255–258, 1993b. Platt, J. P. and Passchier, C. W.: Zipper junctions: A new approach to the intersections of conjugate strike-slip faults, Geology, 44, 10, 795–798, 2016. Russell, P. C., Sorg, D. H. And McLaughlin, R. J.: K-Ar ages of mineralization associated with the San Andreas fault system in west-central California, Bulletin of Isotopic Geochronology, 54, 19–20, 1989. Sylvester, A. G. and Smith, R. R.: Tectonic Transpression and Basement-Controlled Deformation in San Andreas Fault Zone, Salton Trough, California, AAPG Bulletin, 60, 12, 225–299, 1976. Van der Woerd, J., Klinger, Y., Sieh, K., Tapponnier, P., Ryerson, F. J. And Mériaux, A.-S.: Long-term slip rate of the southern San Andreas Fault from 10Be-26Al surface exposure dating of an offset alluvial fan, Journal of Geophysical Research, 111, B04407, 2006.